Bioenergy: New Trends in Biofuel Production
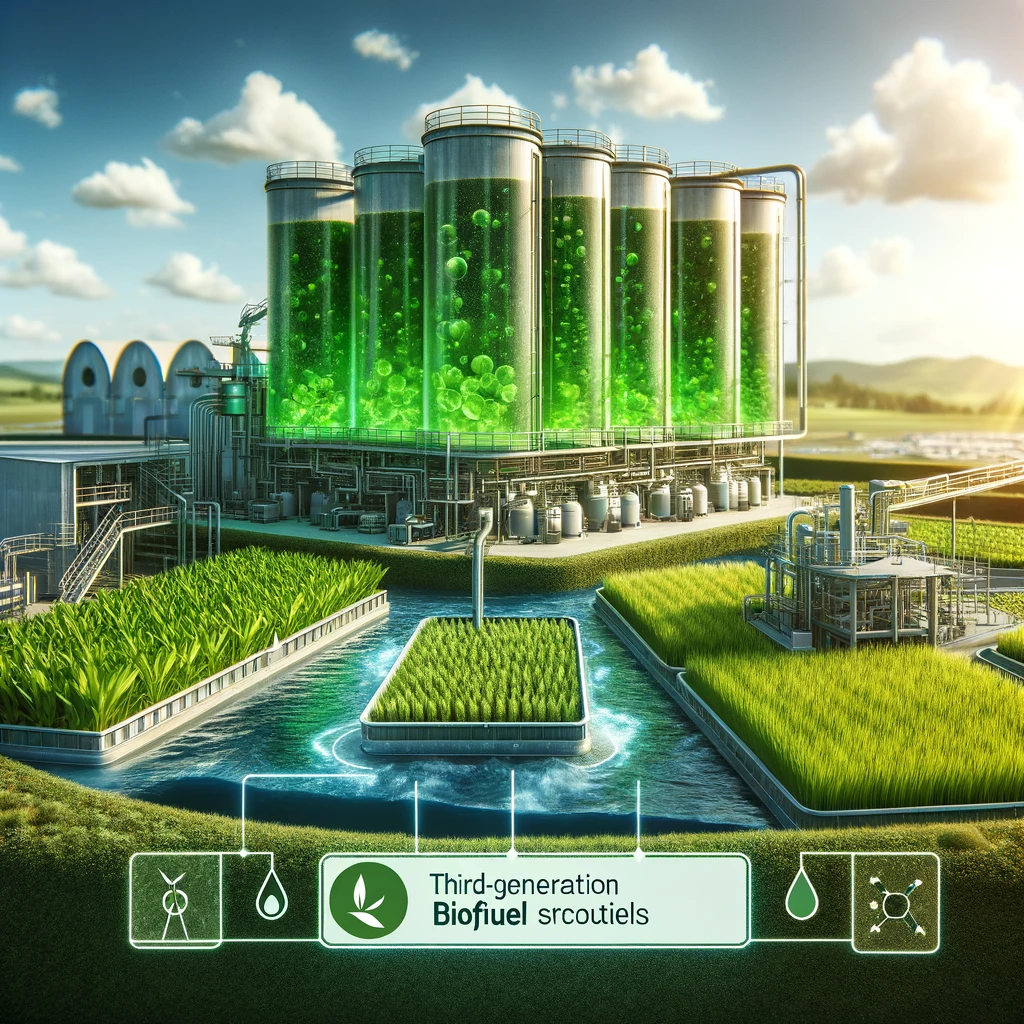
Biofuels, at their core, represent a diverse range of energy sources derived from biomass—organic material from plants and animals. They encompass a wide array of liquid and gaseous fuels, including the most common types such as ethanol, biodiesel, and biogas. These renewable sources of energy are considered a critical alternative to fossil fuels due to their potential to mitigate the effects of climate change, reduce carbon emissions, and foster energy security and sustainability.
The evolution of biofuels is demarcated by generational shifts, each signifying advancements in technology, feedstock options, and sustainability metrics. The first generation of biofuels originated from the conversion of sugars, starches, and oils found in food crops such as corn, sugarcane, soybean, and rapeseed. Ethanol and biodiesel produced from these sources enjoyed early commercial success, benefitting from existing agricultural practices and relatively straightforward conversion processes. However, as these biofuels gained prominence, they triggered the “food vs. fuel” debate, a concern stemming from their reliance on crops otherwise available for human and animal consumption, and their potential to drive up food prices and compete for arable land.
This contention accelerated the pursuit of second-generation biofuels, which shifted the focus to non-food biomass sources, such as wood chips, grasses, agricultural residues, and other types of cellulosic material. The primary allure of these biofuels was their sustainable nature, circumventing the need for land and resources that could otherwise be allocated to food production. While second-generation biofuels addressed some ethical and resource allocation concerns, they introduced new challenges. The technologies for converting cellulosic biomass to fuel were more complex and costly compared to their first-generation counterparts. Moreover, the pre-treatment and enzymatic breakdown of lignocellulosic materials required for the production of these biofuels were energy-intensive and required further innovation to become economically viable at scale.
The quest for even more sustainable and efficient biofuel sources has given rise to the third generation, with algae and waste materials emerging as the focal feedstocks. Algae, in particular, represents a paradigm shift in biofuel production. Unlike land-based crops, microalgae have several compelling characteristics that position them as a superior alternative. They boast a rapid growth rate and high per-acre yield of oil, which can be up to 100 times more than that of soybeans, a common first-generation feedstock. Algae can be cultivated in a variety of environments, including freshwater, brackish water, and marine systems. Importantly, algae can thrive in habitats unsuitable for agriculture, such as non-arable land and water bodies unsuitable for drinking or irrigation. This versatile adaptability opens up opportunities to use locations and water resources that do not compete with food production.
In addition to their prolific oil production capabilities, algae also present a more neutral carbon footprint. They can absorb carbon dioxide as a growth nutrient, thereby capturing greenhouse gases that would otherwise contribute to global warming. This carbon dioxide can come from industrial emissions, potentially turning a waste product into a valuable resource for biofuel production.
Waste materials, another cornerstone of third-generation biofuels, offer an environmentally sound means of producing energy. By converting agricultural residues, urban waste, and other organic by-products into biofuel, this approach not only provides a renewable energy source but also addresses waste management challenges. It aligns with the principles of a circular economy, wherein waste materials are re-purposed, contributing to environmental sustainability and resource conservation.
The transition to algae and waste materials for biofuel production has been necessitated by a convergence of factors—increased awareness of climate change, the limitations of first and second-generation biofuels, and the urgent need to transition away from fossil fuels. These imperatives have spurred scientific research and technological innovations focused on making the production of third-generation biofuels more efficient, cost-effective, and scalable. Advances in biotechnology, genetic engineering, and process optimization continue to push the boundaries of what is possible in biofuel production, setting the stage for algae and waste materials to become key players in the quest for sustainable energy.
The Rise of Algae as a Biofuel Source
As the vanguard of third-generation biofuels, algae present a transformative potential for renewable energy production. The advantages of utilizing algae stem from its exceptional qualities, distinguishing it from earlier generations of biofuels and solidifying its role as a sustainable powerhouse.
The primary advantage that positions algae at the forefront of biofuel innovation is its high oil yield. Algal species, particularly microalgae, are capable of producing large amounts of lipids or oils, which can be processed into biodiesel. These yields surpass those of traditional biofuel crops by a significant margin: for example, algae can produce between 2,000 to 5,000 gallons of biofuel per acre per year compared to soybeans, which yield approximately 50 gallons on the same land area. This high productivity means that less land is required to produce equivalent amounts of biofuel when compared to crops such as corn or sugarcane, reducing the pressure on arable land and minimizing competition with food production.
Sustainability is another compelling advantage of algae-based biofuels. Algae can be cultivated in ponds, photo-bioreactors, or even waste streams, including municipal and agricultural wastewater, thus contributing to water purification while simultaneously growing energy crops. This dual function highlights a significant sustainability edge, as it addresses two environmental challenges at once: biofuel production and waste treatment. Algae cultivation also presents a lower water footprint compared to traditional agriculture since the water can be recycled through closed-loop systems.
Furthermore, algae have a rapid growth rate and can be harvested daily, providing a continuous source of biomass for biofuel production. This contrasts sharply with seasonal crops, which often have a single harvest period each year. The ability to produce a constant supply of biofuel feedstock can lead to more stable and reliable biofuel production chains.
Algae’s minimal land requirement is yet another sustainability feature. Given the flexibility of algae to grow in diverse environments, including deserts and other non-arable regions, it does not encroach on fertile agricultural land. This aspect is crucial, as it alleviates the ethical dilemma of allocating valuable land resources between food production and energy crops.
The cultivation of algae is undergoing continuous innovation, with technologies evolving to maximize efficiency and minimize costs. There are two primary methods of cultivation: open pond systems and closed photo-bioreactors. Open ponds are the most straightforward and cost-effective method, relying on natural sunlight for photosynthesis, and are usually the choice for large-scale operations. However, they are more susceptible to contamination and less efficient in controlling the growing conditions.
In contrast, closed photo-bioreactors offer a controlled environment for algae growth, safeguarding against contaminants and providing the possibility to tailor conditions such as light intensity, temperature, and pH levels to optimize productivity. Though more expensive to install and operate, photo-bioreactors can result in higher yields and faster growth rates, making them an attractive option for locations with less suitable climates or for producing high-value strains of algae.
Once cultivated, the extraction of algal oil for biofuel production is a crucial step. The process often begins with dewatering the algae biomass, followed by cell disruption to release the oils. Various methods are employed for this purpose, including mechanical pressing, ultrasonication, and solvent extraction. Mechanical pressing is similar to traditional oil extraction from seeds, but can be less efficient. Ultrasonication uses sound waves to break down cell walls, while solvent extraction involves using chemicals such as hexane to dissolve the oils, which are then separated and recovered.
To enhance the efficiency of the oil extraction process, recent advancements have been made in the field of genetic engineering and metabolic engineering of algal strains. By tweaking specific pathways, scientists aim to increase the lipid content within algal cells or to produce strains that can easily release their oils without the need for intensive processing. This biotechnological approach aims to reduce the overall energy input and cost, thereby improving the economic feasibility of algae-derived biofuels.
Another innovative area of research is the integration of algae biofuel production with carbon capture technologies. Algae require carbon dioxide for photosynthesis, and they can use the CO2 emissions from industrial processes as a feedstock. This has dual benefits: it helps mitigate carbon emissions by effectively recycling industrial waste gases, and it provides a cost-effective source of carbon for algal growth, further enhancing the sustainability profile of algae biofuels.
The versatility of algae is underscored by the various end-products that can be generated. Apart from biodiesel, algal biomass can be converted into bioethanol, biogas, biohydrogen, and even jet fuel, offering a wide array of bioenergy options. The extraction processes for these fuels are also undergoing innovative changes, with a move toward more energy-efficient and less chemically intensive techniques.
In conclusion, while challenges remain in scaling up and reducing the costs associated with algae cultivation and processing, the current trajectory of technology development is promising. The symbiosis of sustainability and high yield positions algae as a potential cornerstone in the future of renewable energy. The ongoing advancements in cultivation and extraction techniques are paving the way for algae to make the leap from a promising research subject to a commercially viable source of biofuel. With continuous improvement and investment, algae-based biofuels have the potential to become an integral part of a diversified and sustainable energy portfolio.
Utilizing Waste Materials for Biofuel Production
In the quest for sustainable energy sources, the transformation of waste materials into biofuel has emerged as a pivotal strategy. The range of waste streams that can be repurposed into biofuel is extensive, spanning from agricultural residues to urban waste and industrial by-products. Not only does this approach contribute to waste reduction and management, but it also provides a method to generate energy with lower environmental impact compared to traditional fossil fuels.
Agricultural residues are one of the most abundant sources of organic waste suitable for biofuel production. These include plant parts that are not consumed, such as straw, husks, and cobs from cereal crops, bagasse from sugarcane processing, and nutshells from the food processing industry. These materials are primarily composed of cellulose, hemicellulose, and lignin – the building blocks for second-generation biofuels like cellulosic ethanol. Unlike first-generation biofuels, which utilize the edible parts of crops, the use of non-food biomass avoids the ethical issue of competing with food supply.
Urban waste, particularly municipal solid waste (MSW), also holds significant potential for biofuel production. MSW includes a wide range of materials such as food waste, paper, and yard clippings, much of which can be categorized as biodegradable organic matter. Through processes like anaerobic digestion, this fraction of MSW can be converted into biogas, a mixture of methane and carbon dioxide. Biogas can be further purified to biomethane, which is interchangeable with natural gas, and can be used for heating, electricity generation, or as a transportation fuel in the form of compressed natural gas (CNG).
Industrial by-products, such as glycerol from biodiesel production or black liquor from the paper manufacturing process, represent another waste stream that can be redirected towards biofuel generation. Glycerol can be processed through microbial fermentation to produce a range of products including bioethanol, while black liquor contains lignin which can be gasified to produce syngas – a precursor for the production of liquid biofuels.
The processing of these varied waste materials into biofuels involves multiple technologies. Thermochemical processes such as pyrolysis and gasification can convert lignin-rich biomass into syngas. Syngas is a versatile intermediate and can be further processed into liquid fuels like methanol or synthetic diesel through the Fischer-Tropsch process. Alternatively, biochemical conversion processes, including fermentation and anaerobic digestion, are employed to convert the sugar and starch in biomass to bioethanol and biogas, respectively.
The environmental benefits of deriving biofuels from waste materials are multifaceted. Firstly, it diverts waste from landfills, reducing the associated emissions of methane, a potent greenhouse gas. The production and use of waste-derived biofuels also generally emit fewer pollutants, such as sulfur oxides and particulates, compared to fossil fuels, contributing to improved air quality.
An important advantage of waste-derived biofuels is the reduction in life-cycle greenhouse gas (GHG) emissions. By utilizing waste streams that would otherwise decompose and release GHG, these biofuels can achieve a negative carbon footprint under certain conditions. This is particularly true when the alternative fate of the waste is uncontrolled decomposition or incineration without energy recovery.
Moreover, the use of waste materials for biofuel production aligns with the principles of a circular economy, where waste is minimized, and materials are reused, thus prolonging their lifecycle. This approach stands in stark contrast to the linear “take, make, dispose” model, which is resource-intensive and unsustainable.
Another environmental advantage includes the conservation of water and land resources. Unlike traditional energy crops that require significant amounts of water and arable land, waste-derived biofuels utilize materials that are already produced as a by-product of other processes. This not only preserves precious water resources but also prevents the expansion of agricultural land at the expense of natural habitats.
When it comes to the contribution to waste management strategies, the integration of biofuel production into waste treatment facilities can be seen as a modern alchemy, turning what is traditionally seen as valueless refuse into valuable energy carriers. This can significantly reduce the economic and environmental costs associated with waste disposal and treatment, while at the same time producing a tangible energy commodity.
To maximize the benefits of waste-derived biofuels, it is important to optimize the collection, separation, and processing of waste streams. Advanced sorting technologies, pre-treatment methods to improve feedstock quality, and the development of more efficient microbial strains or catalysts for conversion processes are all areas of ongoing research that can enhance the viability and attractiveness of this approach.
The flexibility of waste-to-energy conversion technologies allows for localized solutions to waste management. Small-scale biogas plants can be particularly beneficial for rural areas, providing a source of energy while dealing with agricultural waste on-site. Urban areas, with their dense populations producing large amounts of MSW, can utilize centralized facilities to process waste into biofuel, thus reducing transport costs and emissions associated with waste management.
While the environmental benefits are clear, it is essential to ensure that waste-derived biofuels are produced in a manner that respects biodiversity and does not lead to unintended environmental degradation. For instance, some energy crops can become invasive species if not managed properly, and the collection of agricultural residues must be carried out in a sustainable manner to avoid soil depletion.
Ultimately, the utilization of waste materials for biofuel production represents a key element in the transition towards more sustainable energy systems. By capitalizing on the untapped energy potential of waste streams, we can simultaneously address the challenges of waste management, reduce greenhouse gas emissions, and diminish our dependence on fossil fuels. The continued evolution and integration of waste-to-energy technologies are essential to fully realize these environmental benefits and to contribute to a more sustainable and resilient energy future.
Environmental Impacts of Third-Generation Biofuels
Delving into the environmental impacts of third-generation biofuels, particularly those derived from algae and waste materials, offers a compelling glimpse into the future of sustainable energy. Unlike their predecessors that rely on food crops (first-generation) or lignocellulosic biomass (second-generation), third-generation biofuels promise a reduced ecological footprint while still providing a scalable renewable energy source. However, the environmental narrative surrounding these biofuels is not devoid of complexities and potential trade-offs.
Positive Environmental Impacts
- Lower Greenhouse Gas Emissions: The lifecycle greenhouse gas (GHG) emissions from third-generation biofuels are significantly lower compared to fossil fuels. Algae, for instance, absorb carbon dioxide during photosynthesis, effectively sequestering carbon as they grow. When algae-based biofuels are consumed, the released CO2 is roughly equivalent to what was absorbed, making the process close to carbon-neutral. Waste-derived biofuels further reduce emissions by diverting organic waste from landfills, where it would decompose anaerobically and produce methane, a potent GHG.
- Minimal Land Use: Algae can be grown in non-arable land, including deserts or areas unsuitable for agriculture, using saltwater or even wastewater. This drastically reduces the competition for land with food crops, mitigating the risk of deforestation and preserving biodiversity. Waste-to-biofuel processes do not require additional land as they utilize existing waste streams.
- Water Efficiency: Algae cultivation systems can be designed to utilize wastewater, providing a dual function of water purification and biofuel feedstock production. This reduces the demand for freshwater, a significant advantage over conventional bioenergy crops which can be water-intensive.
- Nutrient Recycling: When grown in wastewater, algae can assimilate nutrients such as nitrogen and phosphorus, which are common pollutants in water bodies. This process of bioremediation not only produces valuable biomass but also helps in restoring the health of aquatic ecosystems.
- Biodiversity Protection: Algal cultivation for biofuel does not necessitate the use of pesticides or herbicides that can harm non-target species. Additionally, by providing an alternative to fossil fuels, which are often extracted from ecologically sensitive areas, third-generation biofuels can play a role in reducing environmental disturbances caused by drilling and mining activities.
Negative Environmental Impacts and Concerns
- Energy Balance: One of the main concerns is the energy balance of biofuel production, particularly the energy return on investment (EROI). For the process to be sustainable, the energy produced from the biofuel must exceed the energy consumed in producing it. Currently, some algae-to-biofuel processes are energy-intensive, particularly during harvesting and oil extraction, which can negate the environmental benefits.
- Land and Water Pollution: Large-scale cultivation of algae, if not managed properly, can lead to nutrient runoff, causing eutrophication in nearby water bodies. While closed photobioreactors limit this risk, they are more costly compared to open pond systems. Additionally, the accumulation of residual biomass post-extraction may create disposal issues, potentially leading to land and water pollution if not handled responsibly.
- Water Consumption: Even though algae can grow in wastewater, the scaling up of production might still require substantial amounts of water, particularly in open pond systems susceptible to evaporation. The impact on water resources could be significant if freshwater is used extensively.
- Habitat Disruption: Establishing large-scale algae farms, even on non-arable land, could lead to habitat disruption. For waste-derived biofuels, the collection, transportation, and processing infrastructure could also have localized environmental impacts, including habitat fragmentation and increased traffic emissions.
- Invasive Species Risk: Some species of algae have the potential to become invasive if they are not contained, particularly in open pond systems. An unintended release into natural water bodies could disrupt local ecosystems and outcompete native species.
Comparative Lifecycle Analysis
A comprehensive lifecycle analysis of third-generation biofuels, accounting for the entire spectrum of their production and use, often exhibits a favorable comparison with traditional energy sources. The energy required to cultivate algae or process waste materials is typically less than that for extracting, refining, and transporting fossil fuels. Additionally, as technologies advance and efficiencies improve, the carbon footprint and overall environmental impact of biofuel production are expected to decrease further.
Furthermore, unlike the extraction of fossil fuels, which becomes increasingly difficult and energy-intensive as resources deplete, biofuel production from algae or waste is renewable. This means that, with proper management and technological advancements, third-generation biofuels have the potential for ongoing reduction in environmental impacts while fossil fuels move in the opposite direction.
Potential Environmental Concerns with Large-Scale Production
The upscaling of third-generation biofuel production to meet global energy demands is not free from potential environmental concerns. The aforementioned risks of nutrient pollution, habitat disruption, and invasive species become more pronounced at scale. Additionally, the construction of large-scale biofuel processing plants may require significant resources, and their operations may generate pollutants.
The infrastructure necessary for large-scale biofuel production must therefore be designed with environmental impact mitigation in mind. This might involve integration with existing industrial processes, such as locating algae cultivation systems near power plants to utilize flue gas CO2, or using by-products from biofuel production as inputs for other industries, thus promoting a circular economy approach.
To conclude, while third-generation biofuels hold great promise for reducing our carbon footprint and providing a more sustainable energy source, their environmental impacts are nuanced. The benefits of lower GHG emissions, efficient land and water use, and the potential for nutrient recycling are significant. Nonetheless, challenges related to energy balance, potential pollution, water consumption, and habitat disruption require careful management and ongoing technological innovation to ensure that the large-scale production of third-generation biofuels aligns with environmental sustainability goals.
Economic Viability and Market Potential
Building on the nuanced environmental implications of third-generation biofuels, the economic viability of these bioenergy sources is a crucial determinant of their potential to supplement or even replace traditional energy sources. To fully appreciate the feasibility of algae and waste-derived biofuels, it is imperative to dissect the cost factors involved in their production and assess their competitiveness in the broader energy market.
Cost Factors in Producing Third-Generation Biofuels
The production costs of third-generation biofuels are contingent on several key factors, ranging from the feedstock procurement to the bioconversion processes and end-product refinement. For algae-based biofuels, the cost considerations start with the cultivation system. Open pond systems are cheaper to construct but are less controlled and efficient compared to photobioreactors (PBRs), which, though more expensive, yield higher productivity and can prevent contamination and water evaporation.
Harvesting the algae is another cost-intensive step, especially considering the large volumes of water that must be processed to extract the algal biomass. Centrifugation, flocculation, and filtration are common methods, each with its own cost implications. The drying of algae, essential for oil extraction, adds further to the energy and cost requirements.
Oil extraction methods such as mechanical pressing, solvent extraction, or supercritical fluid extraction have varying efficiencies and costs. The energy return on investment (EROI) is a pivotal metric at this stage, as the energy consumed in these processes needs to be outweighed by the energy contained in the biofuel produced.
For waste-derived biofuels, the type of waste material used can significantly impact costs. While using residual biomass from agricultural or municipal sources might be less expensive, the pretreatment and transportation costs can add up. Moreover, the technological processes involved in converting waste to biofuel—whether through fermentation, anaerobic digestion, or gasification—also have cost variations.
The cost factors extend beyond production to include purification and upgrading of the biofuel to meet quality standards, integration into the existing fuel distribution systems, and the cost of adhering to environmental regulations and policies.
Competitiveness in the Energy Market
To evaluate the competitiveness of third-generation biofuels within the energy market, one must consider their price relative to conventional fuels and other renewable energy sources. The market price of crude oil significantly influences the economic attractiveness of biofuels. High oil prices improve the competitiveness of biofuels, making investments more appealing, whereas low oil prices can stifle biofuel market growth.
However, cost-competitiveness also hinges on technological advancements that can drive down production costs. Improved algae yield, more efficient harvesting and extraction techniques, and innovations in conversion processes can all contribute to making third-generation biofuels a more viable economic option. This is where economies of scale come into play: as production volumes increase, unit costs can decrease, potentially making biofuels more competitive.
Economic Barriers and Enablers
One of the foremost economic barriers to the large-scale deployment of third-generation biofuels is the capital investment required to establish production facilities. The high upfront costs for advanced cultivation systems, harvesting equipment, and refining infrastructure pose significant financial risk, particularly in the nascent stages of market development.
Additionally, the biofuel industry faces market uncertainty due to fluctuating policy support and subsidy structures. Subsidies play a critical role in making biofuel production economically feasible, but shifts in political priorities can lead to changes in subsidy regimes, affecting long-term investment decisions.
Conversely, enablers such as government mandates for biofuel blending and carbon pricing can create a more favorable economic environment for biofuels. Policies that internalize the environmental costs of fossil fuels, thus reflecting their true societal costs, can improve the market position of biofuels.
Furthermore, societal factors such as consumer preferences for sustainable products and corporate commitments to reducing carbon footprints can stimulate demand for biofuels. Public acceptance is pivotal, as it can lead to increased willingness to pay a premium for green energy, thereby enhancing the market potential of third-generation biofuels.
Impact of Policy and Societal Factors
Policy initiatives and societal drivers have the power to catalyze or curb the growth of the third-generation biofuel market. Regulatory frameworks that set ambitious targets for greenhouse gas emission reductions can mandate the use of biofuels and incentivize research and development in the sector. Investment in R&D is crucial for overcoming technical hurdles and achieving cost reductions through innovation.
Moreover, the integration of biofuels into energy policies must consider the entire supply chain, from sustainable feedstock production to distribution logistics and end-user applications. Policies that support an integrated approach can streamline the biofuel value chain and reduce inefficiencies, cutting down overall costs.
Societal attitudes towards energy consumption and sustainability also influence the biofuel market. Increasing awareness of climate change impacts and the desire for energy independence can lead to more supportive public opinion, translating into market demand. Public-private partnerships, engagement with stakeholders, and transparent communication about the benefits and challenges of biofuels are essential in shaping a supportive societal context.
In summary, the economic viability of third-generation biofuels is a multifaceted issue. While the production costs present challenges to competitiveness, advancements in technology and supportive policy and societal landscapes hold the potential to tilt the scales in favor of biofuels. As the energy landscape evolves, third-generation biofuels must navigate these economic waters to establish their place as a sustainable energy alternative.
Transitioning to innovative processes, the next section will delve into the technological frontiers of biofuel extraction and refinement. These processes stand at the cusp of revolutionizing the biofuel industry, enhancing the efficiency and yield of fuel production. Through genetic engineering, enzyme-assisted hydrolysis, and thermochemical conversion, the biofuel sector is making strides towards greater economic and environmental viability.
Innovative Processes in Biofuel Extraction and Refinement
Innovative Processes in Biofuel Extraction and Refinement
The biofuel industry is at a transformative juncture where emerging technologies in extraction and refinement are beginning to address the sustainability and economic feasibility challenges that third-generation biofuels face. Notably, these breakthroughs focus on improving the conversion of algal and waste biomass into fuel, optimizing production systems for greater efficiency, and reducing the overall environmental footprint of biofuel manufacturing. This exploration will delve into the spectrum of advancements, both incremental and radical, that stand to revolutionize biofuel production processes.
Genetic Engineering of Algae for Enhanced Biofuel Production
One of the most promising areas of innovation is genetic engineering, which can significantly improve the viability and performance of algae as a biofuel source. Through genetic manipulation, scientists are developing algal strains with higher lipid content and growth rates. By isolating and modifying genes responsible for photosynthesis, lipid synthesis, and stress resistance, researchers are creating super strains of algae that not only produce more oil but also thrive in a variety of conditions, reducing the need for stringent environmental controls.
A particularly exciting development is the use of CRISPR-Cas9 gene-editing technology to enable precise modifications in algal genomes. This tool has allowed for the knockout or insertion of specific genes, paving the way for algae to be programmed for optimal biofuel production. Enhanced strains can be tailored to produce specific types of lipids that are more easily converted into biofuels, or that have desirable properties, such as higher energy density or improved cold flow characteristics.
Enzymatic Hydrolysis: Breaking Down Biomass Efficiently
The conversion of waste biomass into biofuels has seen remarkable improvements with the advent of enzyme-assisted hydrolysis. This process involves using specific enzymes to break down complex carbohydrates in biomass into simple sugars, which can then be fermented into biofuels. Enzymes offer a targeted, energy-efficient means of breaking down cellulosic and lignocellulosic materials that make up much of the waste biomass.
Advancements in biotechnology have led to the creation of enzyme cocktails that are tailored to the specific composition of different waste streams. Such precision not only improves the efficiency of the biomass conversion process but also lowers the cost by reducing the enzyme quantities required. Enzymatic hydrolysis conducted at moderate temperatures and pressures further underscores the sustainability of this approach, as it demands significantly less energy compared to traditional thermochemical methods.
Thermochemical Conversion: Innovations in Pyrolysis and Gasification
In the realm of thermochemical conversion, pyrolysis and gasification processes are being fine-tuned to maximize biofuel yields and minimize waste. Pyrolysis involves heating biomass in the absence of oxygen to produce bio-oil, which can be refined into biofuel. Advances in reactor design and process integration have led to the development of fast and flash pyrolysis techniques, which offer quicker bio-oil production rates and enhanced bio-oil quality.
Gasification, on the other hand, converts biomass into syngas, a mixture of carbon monoxide and hydrogen that can be transformed into a range of fuels and chemicals. Innovations in this area include the introduction of catalytic gasifiers that improve syngas quality and yield. Furthermore, advancements in syngas cleaning and conditioning technologies are essential for producing biofuels that meet the required standards for use in transportation and other applications.
Catalytic Conversion: A Leap Forward in Biofuel Refinement
Catalytic conversion technologies stand as a central pillar in the bid to make third-generation biofuels more economically competitive. Scientists are developing novel catalysts that can efficiently process bio-oils and syngas into finished biofuels. These catalysts, often composed of heterogeneous materials, are engineered to withstand the rigors of biomass conversion, providing enhanced activity, selectivity, and stability.
One of the notable breakthroughs is the use of zeolites and metal-organic frameworks (MOFs) as catalysts. These materials offer high surface areas and tunable pore structures that facilitate the conversion of biomass into a broader range of fuel products. The application of these advanced catalytic materials has shown promise in producing biofuels with improved cetane numbers and lower sulfur content, which are crucial for meeting diesel fuel specifications.
Solar Bioreactors: Harnessing the Sun’s Energy for Biofuel Production
The integration of solar energy into biofuel production processes has given rise to solar bioreactors. These systems use sunlight not only to drive the photosynthesis of algae but also to provide the heat required for biofuel extraction and processing. By coupling solar thermal and photovoltaic technologies with bioreactor design, these systems capture solar energy more efficiently and reduce the carbon footprint of biofuel production.
Recent developments in solar bioreactor technology have focused on optimizing light penetration and distribution within the cultivation medium, as well as enhancing thermal management to maintain optimal growth conditions for the algae. Innovations such as the use of light-diffusing fibers and photonic crystals are being explored to maximize the utilization of solar energy and promote uniform algal growth.
Biorefinery Approach: Maximizing Value from Biomass
The biorefinery concept represents a holistic approach to biomass utilization, aiming to extract the maximum value from every part of the feedstock. In a biorefinery, various technologies are integrated to produce not just biofuels but also a spectrum of high-value co-products such as chemicals, materials, and nutraceuticals. This approach improves the overall economics of biofuel production by creating additional revenue streams.
Recent strides in biorefinery development include the use of systems engineering and process modeling to optimize the conversion pathways for different biomass feedstocks. Innovations in separation technologies, such as membrane filtration and supercritical fluid extraction, are being employed to isolate and purify valuable co-products from the biofuel production process. By leveraging these advancements, the biorefinery model enhances the economic viability of third-generation biofuel production, making it a more attractive and sustainable option for investors and policymakers.
Automation and AI in Biofuel Production
The introduction of automation and artificial intelligence (AI) into biofuel production processes represents a leap towards enhanced efficiency and process control. Automated systems equipped with sensors and control algorithms can continuously monitor and adjust parameters like temperature, pH, and nutrient levels to optimize algal growth and biomass yield.
AI, through machine learning algorithms, is being employed to analyze vast datasets generated by these automated systems to identify patterns and optimize production processes. Predictive models can forecast yields, suggest optimal harvest times, and even predict the impact of environmental changes on biofuel production. This technological convergence holds the potential to drastically increase the precision and reduce the costs of biofuel production operations.
Advanced Fermentation Techniques: Pushing the Limits
Fermentation is a critical step in the biofuel production chain, particularly in the conversion of sugars to ethanol or other biofuels. Advances in metabolic engineering and synthetic biology are leading to the development of “designer” microbes that have enhanced fermentation capabilities. These genetically engineered yeast and bacterial strains can ferment a wider range of sugars, including those derived from the hemicellulose and lignin fractions of biomass, thus improving the efficiency of biofuel production.
Economic and Environmental Enhancement Through Water Recycling
In algae cultivation and subsequent biofuel processing, water use is a key sustainability concern. Innovations in water recycling and treatment technologies, including advanced filtration and bioremediation systems, are crucial for reducing water consumption. These systems enable the reuse of process water, minimizing freshwater inputs and reducing the biofuel production footprint. Coupled with the integration of wastewater treatment within algal cultivation systems, this advancement also contributes to wastewater management, further reinforcing the environmental credentials of third-generation biofuels.
Life Cycle Analysis: Ensuring a Net-Positive Impact
Beyond the direct technological innovations, the assessment of biofuel production through life cycle analysis (LCA) has become increasingly important. LCA tools help measure the environmental impacts of biofuel production from cradle to grave, enabling producers to identify and mitigate negative impacts. This holistic approach ensures that each innovation contributes positively to the overall sustainability profile of biofuels, from resource extraction and material processing to end-of-life stages.
Intellectual Property and Collaboration: Protecting and Sharing Innovation
The rapid pace of innovation in biofuel technologies underscores the importance of intellectual property protection as a driver for continued investment and research. Patents and licensing agreements protect breakthroughs and incentivize further development. At the same time, industry collaborations, research consortia, and open-source platforms are emerging as vital spaces for sharing knowledge and fostering innovation, speeding up the transfer of these technologies from the laboratory to commercial-scale production.
By navigating and embracing these innovative processes, the biofuel industry is forging a path towards greater sustainability and economic feasibility. The technologies discussed reflect an ongoing evolution—a symbiosis of biology, chemistry, engineering, and data science—that is shaping the future of energy. Through these advancements, third-generation biofuels are steadily progressing towards a state of increased market readiness, signaling a transition towards more resilient and environmentally harmonious energy systems.
Moving on from the realm of innovation, it is also critical to acknowledge and address the challenges and limitations that exist within the current biofuel technologies. These hurdles, which span from the inherent energy intensity of certain production processes to technical and scalability issues, are crucial focal points for ongoing research and development efforts, as they hold the key to mainstreaming third-generation biofuels and truly capitalizing on their potential to transform the energy sector.
Challenges and Limitations in Current Biofuel Technologies
Challenges and Limitations in Current Biofuel Technologies
As the biofuel industry strives to harness third-generation biofuels as a cornerstone of sustainable energy, it confronts several technical and infrastructural challenges that stymie their widespread adoption. Despite the innovative processes in extraction and refinement, the industry must grapple with intricate hurdles ranging from cultivation dilemmas to economic and infrastructural constraints. The journey to market maturity for algae-based biofuels, in particular, presents a multifaceted set of obstacles that need to be meticulously navigated.
Cultivation Challenges
Algal cultivation, while promising on paper, faces practical complexities. The control of algal cultures on a large scale to prevent contamination and maintain desired strains is a technical challenge. Wild types of algae or invasive species can outcompete the engineered or selected strains, leading to reduced lipid content and compromised biofuel quality. In outdoor ponds, maintaining optimal growing conditions year-round is difficult; temperature fluctuations, light availability, and weather events can drastically impact productivity.
Moreover, the current productivity of algae does not yet meet commercial expectations. The theoretical lipid yields from microalgae have not been fully realized in practice, which is a significant barrier to their commercial viability as biofuel feedstock. High-density cultivation systems that increase yields also come with heightened risks of culture crashes due to pathogens, oxygen depletion, or nutrient imbalances.
Harvesting and Extraction Complexities
The harvesting and extraction phase presents another array of technical challenges. Microalgae harvesting, due to the small size and low concentration of the cells in aqueous cultures, requires energy-intensive processes such as centrifugation or flocculation, which can represent up to 20-30% of the total biofuel production cost. Similarly, the extraction of lipids from algal biomass is not straightforward and often necessitates the use of expensive and environmentally harmful solvents. Methods like supercritical fluid extraction that avoid these downsides are currently too costly for large-scale use.
Energy Balance and Process Efficiency
Energy return on investment (EROI) is a critical metric for evaluating the sustainability of biofuel production. Some current algal biofuel processes have an unfavorable EROI, meaning the energy required for producing the biofuel exceeds the energy obtained from it. This imbalance arises from the high energy inputs required for pumping, mixing, and processing in the cultivation and harvesting stages. To be considered a true alternative to fossil fuels, third-generation biofuels must achieve a positive energy balance.
Bioreactor Design and Scale-Up
While closed bioreactors offer greater control over cultivation parameters and reduce the risk of contamination compared to open pond systems, they are far more expensive to build and operate. Scaling up these systems to the extent necessary for meaningful biofuel production amplifies these cost issues. The materials used for bioreactor construction, typically glass or transparent polymers, can be cost-prohibitive when deployed on a massive scale, and the complexity of maintaining sterile conditions in larger systems can be daunting.
Integration with Existing Infrastructures
Infrastructure incompatibility also poses significant challenges. The current energy infrastructure is primarily designed to handle liquid fossil fuels. Third-generation biofuels, particularly those derived from algae, may have different chemical characteristics and behavior in engines, pumps, and pipelines. For instance, bio-oils can be more corrosive or have higher viscosity compared to traditional fuels, which can create issues without modifications to existing infrastructure.
Furthermore, the logistics of integrating a new form of energy into the existing supply chain are complex. Developing new supply chains for collection, conversion, and distribution of biofuels is a monumental task, requiring collaboration across multiple sectors and significant capital investment. Biofuel production facilities also need to be located strategically to minimize transport costs and maximize efficiency, often requiring a rethinking of the geographical layout of energy production centers.
Regulatory and Policy Hurdles
Regulatory frameworks and policy incentives play a crucial role in the development and adoption of new technologies. Inconsistent or inadequate policies can severely hinder the growth of the biofuel sector. Policymakers must navigate the delicate balance of promoting biofuel technologies without creating market distortions or unintended negative consequences, such as the misallocation of subsidies or overreliance on a single feedstock type.
Economic and Commercial Barriers
From an economic perspective, the cost of third-generation biofuel production remains one of the largest hurdles. The higher production costs relative to fossil fuels and even other renewable energy sources limit competitiveness. Without significant cost reductions in algal cultivation, harvesting, and processing technologies, third-generation biofuels will struggle to find a foothold in the energy market. This requires ongoing investment in research and development to drive down costs and improve efficiencies at every stage of the production process.
Moreover, the market for biofuels is influenced by the volatile price of oil. Low crude oil prices can quickly erode the competitive edge of biofuels, making it difficult for producers to plan and invest with confidence. The ability to achieve cost parity or superiority with fossil fuels is a moving target that adds uncertainty to the biofuel investment landscape.
Technological and Scaling Bottlenecks
On the technological front, the integration of advanced biotechnologies into large-scale biofuel production is still in its nascent stages. While genetic engineering and synthetic biology offer pathways to enhance algal strains and fermentation processes, scaling these laboratory successes to industrial levels presents enormous challenges. Technical bottlenecks in maintaining genetically engineered cultures or optimizing fermentation processes at scale impede the consistent quality and quantity of biofuel production.
In addition to the biological aspects, chemical engineering challenges also play a significant role. The design and implementation of efficient conversion processes, such as catalytic cracking or hydrotreating of bio-oils, have yet to be optimized for algae-derived inputs. Similarly, the development of catalysts that are resilient and effective in these conversion processes often lags behind the pace needed for commercial viability.
Environmental Considerations
Finally, environmental considerations are integral to the advancement of biofuels. The large water footprint of algal cultivation systems raises concerns about water scarcity and the potential impacts on local water resources. Strategies to mitigate these impacts, such as using wastewater streams for algal growth, are still under development and are not yet widely implemented. Nutrient runoff from open pond systems can also contribute to eutrophication in surrounding water bodies, an environmental concern that closed bioreactor systems seek to address but at a higher cost.
Additionally, life cycle assessments of third-generation biofuels must continue to evolve to fully account for their environmental impacts, from cultivation to processing, transportation, and use. While the promise of a lower carbon footprint is a major driver for biofuel research, ensuring that this promise translates into reality requires comprehensive and rigorous environmental accounting.
By addressing and overcoming these multifaceted challenges, third-generation biofuels could inch closer to being a mainstay of the global energy landscape. Innovations in cultivation, harvesting, and conversion methods must be matched with infrastructural, policy, and economic solutions to enable a future where these biofuels contribute significantly to reducing greenhouse gas emissions, enhancing energy security, and advancing sustainable development goals.
Future Prospects and Research Directions
Future Prospects and Research Directions
The march towards third-generation biofuels is not just about the technology itself but about the future it envisages—a future where energy is sustainable, renewable, and accessible. Looking to the horizon, the roadmap for these biofuels is paved with intensive research, innovative practices, and an interdisciplinary approach that could potentially revolutionize the energy sector.
Given the pressing imperative to transition to cleaner energy sources, third-generation biofuels stand at the precipice of significant breakthroughs. Multidisciplinary research is key, marrying biology with nanotechnology, chemical engineering with environmental science, to optimize and refine biofuel production processes. Algal biofuels, in particular, benefit from these collaborative efforts, with biologists engineering high lipid content strains and material scientists developing more efficient bioreactors.
Genetic engineering and synthetic biology are expected to remain at the forefront of algae biofuel advancements. The focus is on manipulating the metabolic pathways to enhance lipid accumulation or directly increase the photosynthetic efficiency of algae. Gene editing tools like CRISPR-Cas9 could lead to breakthroughs in algal bioengineering, allowing scientists to create tailor-made algae strains that thrive in specific conditions and produce fuels more efficiently.
Robotics and automation technology are also evolving and are likely to play a role in the future of biofuel production. Automated systems for the continuous monitoring and adjustment of algae cultivation conditions could lead to more consistent yields and lower labor costs. Machine learning algorithms can predict and optimize growth parameters, harvesting schedules, and nutrient dosing to maximize lipid production while minimizing resource input.
Integration of waste streams into biofuel production is an exciting area of development, driven by the need for resource efficiency. Technologies that enable the conversion of various forms of organic waste into biofuels are gaining traction. These include anaerobic digestion for biogas production, gasification, and new forms of enzymatic hydrolysis that break down complex waste organics into fermentable sugars for bioethanol production. The challenge lies in engineering robust systems that can handle the heterogeneity of waste while remaining economically viable.
The role of third-generation biofuels within the larger context of renewable energy is also evolving. Their potential to integrate with other sustainable energy initiatives, like solar and wind power, presents an intriguing proposition. For instance, integrating biofuel production with solar panels, also known as photobioreactors, can offset some of the energy demands of biofuel production processes. Such hybrid systems could open the way for more off-grid and decentralized energy production models, particularly in remote or rural areas where energy access remains a challenge.
Biorefineries are a key concept shaping the future of biofuel production, where the goal is to produce not just biofuels but also high-value bioproducts such as pharmaceuticals, bio-plastics, and nutraceuticals. This integrated approach can improve the overall economics of algae cultivation by tapping into multiple revenue streams. Research is underway to streamline these processes, ensuring they are scalable and can be implemented within existing industrial infrastructures.
Policy development and international collaboration also have a critical role in determining the trajectory of third-generation biofuels. Policymakers must create a stable, supportive framework for biofuel research and commercialization, with adequate funding, clear regulations, and incentives for reducing greenhouse gas emissions. International treaties and collaborative projects can facilitate the exchange of ideas and technologies, accelerating progress and adoption across borders.
The quest for a carbon-neutral biofuel that does not compromise food security or biodiversity has taken researchers into exciting new territories. For instance, using saltwater or wastewater for algae cultivation not only conserves freshwater resources but also enables the remediation of these water bodies. Coupled with the adoption of CRISPR-Cas9 technology for genome editing, the path towards strains of microalgae that can thrive in suboptimal conditions while delivering high yields of biofuel is becoming increasingly clear.
In tandem with biological research, advances in materials science promise to develop more effective and sustainable bioreactor systems. Innovative materials that maximize light penetration and minimize contamination are being investigated. Furthermore, advances in nanomaterials could enhance the catalytic conversion processes necessary for turning algae into usable fuels, making them more efficient and less energy-intensive.
Socioeconomic research must also advance to understand the implications of a large-scale shift to biofuel production. The impact on rural economies, employment, and land use will require careful analysis and planning. Creating a sustainable bioeconomy requires not just technological innovation, but a holistic consideration of social, environmental, and economic factors.
Moreover, advancements in logistics and distribution networks will be needed to ensure the efficient delivery of these biofuels to the end-users. This involves developing new storage and transportation solutions that are compatible with biofuels’ different physical and chemical properties compared to conventional fuels. Investment in infrastructure to support the burgeoning biofuel industry will be crucial for its success.
As research propels forward, the scalability of these technologies remains a hurdle that needs to be overcome. Pilot programs and demonstration plants are crucial stepping stones, providing real-world data on the practicalities of large-scale production. These initiatives offer insight into the challenges of scaling up from the lab bench to industrial levels, including maintaining genetic stability of algae, optimizing cultivation conditions, and ensuring the reliability of harvesting and extraction processes.
The potential for third-generation biofuels to be part of a diversified energy portfolio is significant. They could fill niches that other renewable sources cannot, such as providing liquid fuels for transportation or acting as a backup for intermittent sources like solar and wind. As research continues to break new ground, these biofuels may become a staple in the global quest for energy security and sustainability.
In conclusion, third-generation biofuels represent a dynamic field replete with opportunities for innovation and improvement. The future of these biofuels lies in the ability of researchers, policymakers, and industry leaders to collaborate, overcome technical and economic barriers, and embrace an interdisciplinary approach. As the world grapples with climate change and the urgent need for sustainable energy solutions, third-generation biofuels may just offer the versatility and sustainability to be a part of the answer.
Conclusion: The Road to Energy Sustainability
In the quest for a sustainable energy future, third-generation biofuels derived from algae and waste materials have emerged as a beacon of innovation and possibility. These biofuels promise to pivot the energy sector from its deep-rooted reliance on finite and polluting fossil fuels to a more sustainable, renewable, and cleaner matrix. The potential of algae as a biofuel source is enormous, not just for its high oil yield but also for its ability to flourish in a variety of environments. Algae do not compete with food crops for arable land, thus sidestepping the food-versus-fuel conflict that plagued first-generation biofuels. Cultivation can occur on non-arable lands, utilizing brackish or wastewater, which provides an added environmental benefit by treating these waters while producing energy.
Advances in biotechnology have propelled algae into the forefront of biofuel research. Genetic engineering and synthetic biology approaches aim at optimizing their inherent properties to enhance lipid accumulation and improve photosynthesis efficiency. Coupled with these biological tactics, novel cultivation methods, such as photobioreactors that make use of sunlight, are reducing the carbon footprint of biofuel production. The innovative processes do not end at cultivation; breakthroughs in extraction and refinement technologies have also demonstrated a leap in efficiency. Emerging techniques like the use of nanomaterials and nanocatalysts promise to transform biomass into biofuels more effectively, making the production process less energy-intensive and potentially more cost-effective.
Similarly, the utilization of waste materials for biofuel production capitalizes on a circular economy, turning what would be environmental pollutants into valuable energy sources. Through processes like anaerobic digestion and gasification, different types of waste — from agricultural residues and urban waste to industrial by-products — are being transformed into bioenergy. This approach not only reduces greenhouse gas emissions by diverting waste from landfills but also provides an alternative and potentially cheaper feedstock for biofuel production. It showcases a commitment to waste minimization and resource recovery, which are key principles of environmental sustainability.
The environmental impacts of third-generation biofuels are multi-faceted. By reducing dependence on fossil fuels, these biofuels could significantly cut down the greenhouse gas emissions responsible for climate change. Moreover, the lower land and water footprints associated with algae and waste material-based biofuels alleviate the pressures on these critical resources. Despite these advantages, some environmental concerns, such as the preservation of biodiversity and the energy balance of production processes, require continuous monitoring and improvement. Striving for a net positive environmental impact is crucial, and research in this area remains vital.
Economically, the viability of third-generation biofuels hinges on the balance of production costs against the scalability and market demand. Currently, the production of biofuels from algae or waste materials is more expensive than fossil fuels. However, this can change with technological advances, increased scale, and supportive policy frameworks that incentivize sustainable energy practices. Market potential for biofuels also depends on consumer acceptance and the development of infrastructure compatible with biofuel distribution and usage. Here, both government action and private investment play pivotal roles, influencing the rate at which these biofuels can compete in the market.
Furthermore, as we march towards a comprehensive energy portfolio, biofuels may occupy niches that other renewables cannot. They provide a promising solution for liquid fuel needs in transportation and can serve as a stable backup for intermittent sources like solar and wind energy. This complementary role in the energy mix underscores the importance of biofuels in the long-term strategy for energy security and diversification.
In the broader landscape, third-generation biofuels exemplify the collaborative efforts needed across various disciplines and sectors. From biological research to materials science, and from economics to policy-making, progress in this field is inherently reliant on interdisciplinary collaboration. Sharing knowledge and technology across borders enhances the speed of innovation, helping to overcome existing challenges and paving the way for global adoption of these sustainable fuels.
Despite their promise, third-generation biofuels are not without their challenges. Energy-intensive production processes, scalability issues, and the integration of biofuels with current infrastructure represent significant hurdles. These obstacles are not insurmountable but require concerted effort and dedication to innovation, policy-making, and international collaboration.
In reflection, the evolution of biofuel technology epitomizes human ingenuity and the ongoing quest for sustainability. The advances made thus far in algae and waste material-based biofuels are stepping stones towards a cleaner, renewable energy landscape. By harnessing the potential of these resources, the energy sector can move closer to its sustainable goals, reducing its ecological footprint while offering economic opportunities. As research continues to surmount the technical and economic barriers, these biofuels edge ever closer to becoming a key player in the global endeavor for energy security and environmental preservation.
The journey towards a sustainable energy future is an ongoing process, but one that holds great promise, particularly through the lens of third-generation biofuels. Embracing the collaborative spirit of innovation and the concerted push towards eco-friendly alternatives to fossil fuels, the road ahead is one of exciting potential and profound impact. Algae and waste materials have taken center stage in this venture, representing not only a sustainable source of energy but also a symbol of the possibilities that arise when environmental consciousness and technological innovation converge.