CRISPR and Agriculture: Revolutionizing Crop Engineering
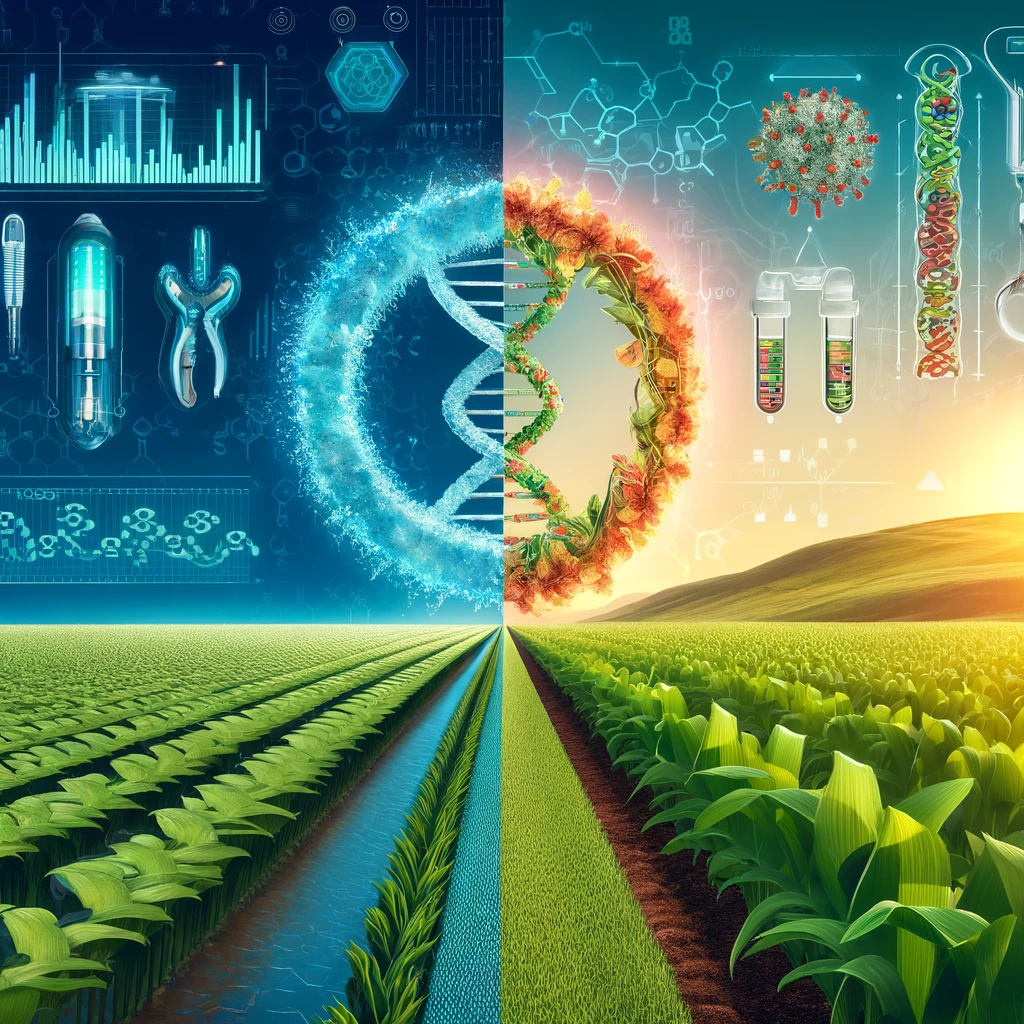
CRISPR technology, an abbreviation for Clustered Regularly Interspaced Short Palindromic Repeats, is a groundbreaking tool that has revolutionized the field of genetic engineering. This technology, derived from a natural defense mechanism found in bacteria and archaea, allows for precise and efficient editing of genetic material, a capability that has far-reaching implications for agriculture. The origins of CRISPR technology can be traced back to observations made by scientists in the 1980s and 1990s when they noted the presence of peculiar repeated DNA sequences in the genomes of bacteria. These sequences were later identified as part of a sophisticated adaptive immune system used by these microorganisms to fend off viral infections.
At its core, the CRISPR system operates as a molecular scissor, guided by a specifically designed RNA molecule to a particular location in the genome. Here, the Cas9 enzyme, the most commonly used CRISPR-associated protein, produces a precise cut in the DNA. When the cell repairs this break, it does so with introduced changes that either disrupt a gene or fix a mutation, effectively allowing scientists to rewrite the genetic code. This system emulates a sort of genetic word processor, capable of adding, deleting, or altering the letters of DNA with exceptional accuracy.
The CRISPR-Cas9 system, in particular, has gained prominence due to its simplicity, efficiency, and versatility. Unlike previous methods of gene editing, such as zinc finger nucleases (ZFNs) or transcription activator-like effector nucleases (TALENs), CRISPR-Cas9 is more accessible, cost-effective, and can target multiple genes simultaneously, making it the preferred choice for researchers. It has become synonymous with gene editing and has turned into a standard tool in molecular biology laboratories worldwide.
In the context of agriculture, CRISPR presents unprecedented opportunities. The agricultural sector is facing monumental challenges, including climate change, a growing global population, and the increasing demand for sustainable farming practices. CRISPR-Cas9 technology provides a way to develop crop varieties that can meet these challenges by introducing traits such as drought tolerance, disease resistance, and improved nutritional content without relying on traditional breeding methods which are often time-consuming and less precise.
Scientists have successfully used CRISPR to enhance disease resistance in a variety of crops, by either tweaking existing genes or by inserting new genetic sequences that equip the plants with the ability to resist pathogens. For instance, by altering or knocking out specific genes, researchers have created rice strains that are resistant to bacterial blight and wheat varieties that are less susceptible to powdery mildew. Such alterations do not introduce foreign DNA into the plant, which may lead to these crops being more readily accepted by regulators and the public compared to traditional genetically modified organisms (GMOs).
Further exploiting CRISPR’s capabilities, scientists have begun addressing issues of food security by increasing the nutritional value of crops. By editing genes involved in metabolic pathways, crops can be biofortified to produce higher levels of vitamins, minerals, and amino acids, leading to more nutritious food for populations worldwide. This aspect of CRISPR technology not only targets the production of food but also its quality, aligning with global health initiatives.
Moreover, the application of CRISPR technology extends to optimizing plants for changing environmental conditions. As global temperatures rise and weather patterns become more unpredictable, there is a pressing need for crops that can withstand such stresses. Through precise genetic modifications, crops are being engineered to tolerate extreme temperatures, saline soils, and limited water availability. Such improvements are essential for maintaining crop yields and ensuring food security in the face of climate change.
CRISPR technology has thus positioned itself at the forefront of agricultural innovation. As we unlock the full potential of the CRISPR-Cas9 system, and explore further advancements like base editing and prime editing, the prospects for sustainable and resilient agriculture only grow brighter. By enabling the creation of crops with fine-tuned genetic traits, CRISPR paves the way for an era of agriculture that is more productive, environmentally friendly, and better equipped to meet the demands of the future.
Scientific Advancements in CRISPR and Agriculture
Since the discovery of CRISPR and its potential for precise genetic modification, a series of scientific advancements have rapidly propelled the technology to the forefront of agricultural innovation. Researchers have made significant strides in developing new CRISPR systems, honing the precision of these genetic tools, and creating success stories by engineering resistance traits in crops. These advances not only hold the promise of revolutionizing how we approach plant breeding but also serve as a testament to the ingenuity of modern science.
One of the key advancements that paved the way for CRISPR’s application in agriculture was the development of new and varied CRISPR systems. While CRISPR-Cas9, derived from Streptococcus pyogenes, is the most renowned and widely used system, scientists have unearthed alternative CRISPR systems with unique properties. For instance, the CRISPR-Cpf1 (Cas12a) system, sourced from Prevotella and Francisella bacteria, offers a different PAM (Protospacer Adjacent Motif) recognition sequence, allowing researchers to target regions of the genome previously inaccessible to Cas9. Additionally, Cpf1 cleaves DNA in a staggered pattern rather than a blunt cut, which may facilitate more efficient integration of desired genetic material.
The discovery of CRISPR-Cas13, an RNA-targeting CRISPR system, has broadened the scope of genetic editing. Unlike Cas9 and Cpf1, which target DNA, Cas13 specifically targets RNA, providing a means to control gene expression without altering the genome itself. This is a critical tool for the study and manipulation of gene function and for developing crops with adjustable trait expression.
Furthermore, improvements in the precision of CRISPR systems have been pivotal in minimizing off-target effects, a major concern in gene editing. Techniques such as high-fidelity Cas9 variants, engineered with mutations to reduce non-specific DNA interactions, have greatly enhanced the accuracy of gene editing. Additionally, the development of base editors — a class of CRISPR-Cas9-derived tools that change individual DNA bases without creating double-strand breaks — has enabled pinpoint modifications, further reducing unintended mutations and providing a more subtle approach to genetic manipulation.
The evolution of prime editing, often referred to as “search and replace” for DNA, marks another leap forward in precision gene editing. Prime editors can be programmed to locate specific genomic sequences and replace them with desired edits without making double-strand breaks or relying on donor DNA templates, thus reducing potential errors associated with DNA repair.
In the realm of agricultural biotechnology, these scientific advancements have led to several success stories where CRISPR has been used to engineer resistance traits in crops. An example of this is the work done on rice — a staple food crop for more than half the world’s population. Researchers have employed CRISPR-Cas9 to target and mutate the OsSWEET13 gene, which is hijacked by the bacterial pathogen causing bacterial blight. Modified rice plants with mutations in this gene have shown increased resistance to the disease without compromising yield.
Another significant success story involves the use of CRISPR to confer resistance to powdery mildew in wheat. By editing the mildew resistance locus (MLO) genes, which when mutated provide resistance to this fungal pathogen, scientists have been able to develop wheat varieties that are less susceptible to powdery mildew, addressing a major challenge in wheat production.
CRISPR technology has also been utilized to combat the devastation caused by viruses in crops such as cassava, an important food source in tropical regions. Using CRISPR-Cas9 to target and disrupt the replication process of the cassava brown streak virus, researchers have created cassava varieties that are showing promise in resisting this damaging disease, potentially saving a critical resource for millions of people.
The ability to enhance crops’ inherent defenses against environmental stresses has been another arena where CRISPR technology shines. For instance, the CRISPR-mediated introduction of drought tolerance in maize and soybean through the editing of native genes involved in water-use efficiency signifies how targeted genetic improvements can increase the resilience of crops to climate variability.
The flexibility of CRISPR technology extends to combating threats posed by parasitic plants such as Striga, which can have a devastating impact on crops like rice, maize, and sorghum in sub-Saharan Africa. By targeting genes related to germination stimulants in host plants, CRISPR-Cas9 can be employed to diminish the Striga seeds’ ability to germinate, thereby preventing them from attaching to and draining resources from the host plants.
These milestones have not only cemented CRISPR as a vital tool in the fight against crop diseases and environmental stresses, but they have also paved the way for developing novel breeding strategies. The speed, efficiency, and precision of CRISPR technologies are setting the stage for a future where agricultural practices can be more responsive to the dynamic challenges of feeding a growing global population sustainably.
Continued research and development in CRISPR technology, therefore, holds great potential for the further enhancement of crop characteristics, including yield, nutritional content, and tolerance to biotic and abiotic stresses. With every new advance in CRISPR technology, scientists are equipped with better tools to tailor crops to the ever-evolving needs of our planet.
Practical Applications of CRISPR in Crop Engineering
CRISPR technology has transitioned from a promising scientific concept to a transformative tool in the agricultural sector. Its applications in crop engineering are vast and diverse, addressing some of the most pressing issues that affect crop yield and farmer practices. As the global population grows, the need for sustainable and resilient agricultural systems becomes ever more crucial, and CRISPR stands at the forefront of meeting these demands.
Case Study: Engineering Rice for Bacterial Blight Resistance
One of the significant milestones achieved using CRISPR technology in crop engineering is the development of rice varieties resistant to bacterial blight. Bacterial blight, caused by the pathogen Xanthomonas oryzae pv. oryzae, is a devastating disease that affects rice crops worldwide, particularly in Asia. The traditional approach to breeding for disease resistance involves crossbreeding rice varieties that naturally possess resistance genes, a process that can take years.
Using CRISPR-Cas9, scientists targeted the OsSWEET14 gene, which encodes for a protein that the bacteria manipulate to gain entry into the plant cells. By knocking out this gene, the pathway for bacterial infection was effectively blocked. The CRISPR-edited rice plants not only exhibited strong resistance to bacterial blight but also retained their yield and quality.
The success of this approach is evident in the field, where these engineered rice varieties provide farmers with a robust defense against a disease that can wipe out their entire crops. This direct genetic intervention has significantly reduced the use of chemical treatments, which has both environmental and economic benefits.
Case Study: Wheat and Resistance to Powdery Mildew
Wheat, another vital staple crop, has been the subject of CRISPR-related studies aimed at improving disease resistance. Powdery mildew, a fungal disease caused by Blumeria graminis, can cause significant yield losses in wheat production. The disease is traditionally controlled using fungicides and through the breeding of resistant varieties, but both methods have their limitations.
CRISPR has been utilized to create wheat lines with mutations in the MLO gene family, which are associated with susceptibility to powdery mildew. Targeted mutagenesis of these genes has led to the development of wheat plants that are more resistant to the disease. Not only does this reduce the dependency on chemical fungicides, which are costly and can have negative environmental impacts, but it also secures a vital food source against a major production threat.
Farmers growing these CRISPR-engineered wheat varieties could potentially benefit from decreased input costs for fungicides and higher yield stability, even in the presence of the disease.
Case Study: Combatting Viral Threats in Cassava
Cassava is a key food crop in many tropical regions but is threatened by viral diseases like cassava brown streak disease (CBSD) and cassava mosaic disease (CMD). Both diseases can lead to complete loss of the tuberous roots, which are the edible part of the plant. Traditional breeding methods for disease resistance have had limited success due to the complex genetics of cassava and the evolution of viral strains.
Researchers have employed CRISPR-Cas9 to confer viral resistance in cassava by targeting the viral genomes or by modifying the host plant’s genome to hinder the virus’s life cycle. In one study, the CRISPR system was used to target and degrade the RNA of the cassava brown streak virus in infected plants, which resulted in significantly reduced viral loads.
The development of disease-resistant cassava plants through CRISPR not only has the potential to improve the livelihoods of smallholder farmers in affected regions but also to enhance food security for millions who rely on this crop.
Case Study: Enhancing Soybean Oil Quality
Soybean is a widely cultivated crop, valued for its oil and protein content. However, the nutritional profile of soybean oil can be improved by reducing the levels of saturated fats and increasing the content of oleic acid, which is beneficial for heart health. Traditional methods to alter the fatty acid composition of soybean oil are time-consuming and often result in unwanted trade-offs.
Using CRISPR-Cas9, scientists have successfully edited the genes involved in the fatty acid synthesis pathway in soybeans. These edits resulted in an increased oleic acid content and decreased levels of saturated fats, leading to an improved oil profile without compromising the plant’s overall fitness.
Farmers cultivating these enhanced soybean varieties can tap into the growing market demand for healthier oils. Additionally, the oil from these soybeans is more stable and has a longer shelf life, which is beneficial for both consumers and the food industry.
Case Study: Developing Drought-Tolerant Maize
Climate change poses a severe threat to crop production, and drought is one of the most critical stress factors affecting maize yield. Traditional breeding for drought tolerance is challenging due to the quantitative nature of the trait, which is controlled by many genes.
CRISPR technology has been harnessed to create drought-tolerant maize by editing genes involved in water-use efficiency and stress response pathways. One such target is the ARGOS8 gene, which regulates plant growth hormones and stress responses. By modifying this gene, researchers have developed maize varieties that maintain higher yields under water-limited conditions.
This advancement is crucial for farmers facing unpredictable rainfall and water scarcity. Drought-tolerant maize varieties can help ensure food security and provide more stable income for farmers in drought-prone regions.
The impact of CRISPR in crop engineering is profound, with each case study serving as a testament to the practical applications and benefits of this technology in agriculture. By addressing specific challenges, from disease resistance to environmental stresses, CRISPR is reshaping agricultural practices and offering hope for sustainable and resilient food systems. As CRISPR technology continues to advance, the potential for further improvements in crop yield and farmer practices remains promising, underpinning its role as a key player in the future of agricultural innovation.
Evaluating the Risks and Ethical Considerations
The introduction of CRISPR technology in agriculture has indeed sparked a revolution, offering previously unattainable levels of precision and speed in crop breeding. However, its rapid adoption and vast potential come with a suite of concerns that touch upon the very fabric of food safety, biodiversity, ethics, and the sanctity of life’s genetic blueprint.
Genetic Diversity and Gene Pool Integrity
A primary concern with gene editing, and CRISPR specifically, is its potential impact on genetic diversity. Genetic diversity within crop species is vital for resilience against diseases, pests, and changing environmental conditions. It acts as a repository for traits that might be needed to adapt to future challenges. However, the ease with which specific traits can be edited might lead to a narrowing of the genetic pool if agricultural practices excessively favor certain genetically engineered varieties.
The adoption of genetically uniform crops over large areas could increase the susceptibility of these crops to widespread disease or pest outbreaks, reminiscent of the Irish Potato Famine in the 19th century. The safeguarding of genetic diversity thus requires a careful and integrated approach to CRISPR editing in crops, ensuring that edited traits complement, rather than replace, the broader genetic pool. This is particularly important in centers of origin and diversity for certain crops, where the range of genetic variation is greatest and should be conserved.
Biosafety and Environmental Concerns
From a biosafety standpoint, the potential for off-target effects, where the CRISPR-Cas9 system might inadvertently alter DNA sequences other than those intended, poses a significant risk. Though advances in the technology have greatly reduced these occurrences, they cannot be entirely ruled out. Unpredicted genetic changes could lead to the emergence of new allergens or toxins, or unintended effects on the crop’s physiology and its interactions with the ecosystem.
Another concern relates to gene flow—the transfer of genes from genetically engineered crops to wild relatives or non-GM crops—which might unintentionally introduce modified traits into the natural environment or neighboring agricultural systems. This gene flow could lead to unforeseen ecological consequences, such as the creation of “superweeds” resistant to herbicides or the disruption of local biodiversity.
Regulatory agencies around the world are grappling with the challenge of evaluating the biosafety of CRISPR-engineered crops. This involves not just examining the end product, but also scrutinizing the process, ensuring that gene editing is precise, safe, and does not produce unintended outcomes that could pose risks to human health and the environment.
Ethical Considerations of Genetic Alteration
The ethics of gene editing in crops stir up deep philosophical and moral questions. Altering the genome of living organisms challenges long-held beliefs about the sanctity of natural life processes. Ethical considerations are not just about the potential risks, but also about the very act of human intervention at the genetic level. Some argue that we are overstepping our bounds as stewards of the Earth by manipulating the genetic essence of life forms for our own purposes.
The ethical debate extends into issues of ownership and control over genetic resources. CRISPR technology raises the question of patenting edited genes and the resulting crops, which can restrict access to these innovations and potentially lead to monopolization by a few powerful corporations. This not only affects farmers and consumer choice but can also have wider implications for food sovereignty and global equity.
In addressing these ethical concerns, it is critical to establish a transparent and inclusive dialogue that involves scientists, ethicists, farmers, policymakers, and the general public. There should be robust frameworks that allow for the responsible and equitable use of CRISPR technology.
Managing Risks and Concerns
To manage these concerns, robust regulatory frameworks and oversight mechanisms are necessary. Effective risk assessment protocols specific to gene editing must be implemented, involving stringent testing and monitoring of CRISPR-modified crops before and after release into the market. Multinational agreements and guidelines, such as those outlined by the Cartagena Protocol on Biosafety, can provide a basis for international standards in the management of genetically modified organisms.
Moreover, the establishment of gene banks and preservation efforts for unmodified varieties is crucial for maintaining genetic diversity. Such repositories can serve as a backup to restore or breed new crop varieties should the need arise. They also play a role in safeguarding indigenous crop varieties that might otherwise be overshadowed by engineered counterparts.
Integrating CRISPR technology into the existing agricultural practices necessitates not only evaluating its immense potential but also responsibly addressing the risks and ethical dilemmas it poses. Striking a balance between innovation and precaution will be paramount in ensuring that the benefits of CRISPR in agriculture can be realized without compromising biosafety, genetic diversity, or ethical integrity. It’s clear that the journey of CRISPR from the lab to the field involves much more than scientific ingenuity; it demands a concerted and multidisciplinary effort to navigate the complex terrain it presents.
Regulatory Framework and Public Perception
Regulatory Framework and Public Perception
The transformation of agriculture by CRISPR technology is not just a scientific or technological issue; it is deeply enmeshed with legal, regulatory, and public opinion landscapes that vary significantly across the globe. Different regions have approached the legal status of CRISPR-modified crops in diverse ways, influenced by scientific evidence, cultural values, ethical beliefs, and public sentiment. This complex interplay significantly affects the development and adoption of CRISPR technologies in the agricultural sector.
Legal Status of CRISPR-Modified Crops
United States: The United States Department of Agriculture (USDA) has adopted a relatively permissive approach to the regulation of CRISPR-modified crops, treating them in a similar manner to traditionally bred plants, as long as they do not contain foreign DNA from plant pests. The USDA’s stance is rooted in a view that the end product—genetically edited crops—is no different from the outcome of natural mutations or traditional breeding techniques. This regulatory environment has been conducive to the research and development of CRISPR-based agricultural innovations.
European Union: In contrast, the European Union has taken a more cautious approach, categorizing CRISPR-modified crops as genetically modified organisms (GMOs) and subjecting them to the same stringent regulatory framework designed for GMOs. This decision came from the European Court of Justice in 2018, which determined that organisms obtained by mutagenesis are GMOs within the meaning of the directives that govern these products. The result has been a more restricted development and release of CRISPR-engineered crops within the EU.
China: China, as a leading nation in CRISPR research, is actively promoting the adoption of gene-edited crops. The country’s Ministry of Agriculture has issued biosafety certificates for CRISPR-modified crops, such as disease-resistant wheat and drought-tolerant rice. The regulatory framework is forward-looking and supportive of innovation, aiming to leverage CRISPR to ensure food security and agricultural development.
Brazil: Brazil, an agricultural powerhouse, has also embraced CRISPR technology by establishing a regulatory distinction between transgenic crops and those edited using new breeding techniques like CRISPR. Brazilian regulations focus more on the process than the product, allowing a streamlined path for CRISPR-edited crops that do not contain foreign genetic material.
These examples demonstrate the varying degrees of legal acceptance and the different regulatory philosophies that inform how countries manage the introduction of CRISPR-modified crops.
Public Acceptance and Ongoing Debates
The role of public perception in influencing regulatory decisions cannot be overstated. Varying levels of acceptance of CRISPR and other gene-editing technologies can be seen across different demographics and regions. For instance, surveys in the United States have shown a general willingness to embrace CRISPR-engineered foods if they offer clear benefits, such as enhanced nutrition or reduced pesticide use. Conversely, in Europe, public skepticism towards genetically modified foods has historically been high, and this extends to gene-edited crops, despite their promise of addressing agricultural challenges.
Public concerns often revolve around issues of safety, ethical implications, environmental impact, and corporate control over the food system. The debate on whether CRISPR-edited crops should be classified and regulated as GMOs or not remains contentious. Some argue for more stringent regulations due to the potential risks that gene editing poses, while others advocate for a science-based approach, emphasizing that gene editing can produce crops indistinguishable from those developed through traditional breeding.
The organic farming community and various advocacy groups have voiced strong opinions against the use of gene editing in agriculture, leading to widespread public discussions. These debates shape public opinion and can thus have a substantial impact on political will and regulatory frameworks.
The public’s role in the CRISPR dialogue is not passive; it actively shapes the direction of policy. Governments and regulatory agencies often engage in public consultation processes to gauge sentiment and include societal values in their decision-making. The case of gene editing highlights the need for transparent communication between scientists, regulators, and the public to build trust and consensus on the future of our food systems.
In the end, the acceptance and implementation of CRISPR-modified crops depend on the complex interplay between science, regulation, public perception, and market dynamics. Balancing innovation with caution, the global community continues to negotiate the path forward for CRISPR in agriculture. The challenge lies in establishing regulatory paradigms that protect public and environmental health without stifling the potential of CRISPR to revolutionize agriculture and contribute to food security and sustainability. As these discussions evolve, they will inevitably contribute to shaping the legal and societal contours within which CRISPR technology will operate in the future.
The Role of CRISPR in Addressing Global Food Security
As CRISPR technology increasingly permeates agricultural practices, its potential to address global food shortages and nutritional deficiencies is becoming more apparent. The implications of this revolutionary technology extend far beyond the realms of scientific research, reaching into the lives of billions who may be affected by food insecurity and malnutrition. The innovation that CRISPR presents lies in its precise and efficient ability to edit genetic material, paving the way for significant advancements in crop production and resilience, which are critical in the face of an escalating global population and the adversities posed by climate change.
A salient implication of CRISPR in agriculture is its capability to enhance crop yields. The modification of genes responsible for growth and yield traits can lead to the development of crop varieties that produce more food per plant. This is vital for feeding the growing population, which is expected to exceed 9 billion by 2050. CRISPR can be employed to amplify desirable traits such as drought tolerance, nutrient use efficiency, and resistance to pests and diseases, all of which contribute to more reliable harvests and bountiful yields.
The enhancement of nutritional content is another significant aspect of CRISPR’s impact on agriculture. With malnutrition affecting large sections of the global population, the ability to biofortify crops using CRISPR holds promise in combating nutritional deficiencies. By tweaking the genetic makeup of staple crops, scientists can increase levels of essential vitamins and minerals. For example, CRISPR has been used to enrich rice with vitamin A, an innovation known as Golden Rice, aimed at addressing vitamin A deficiency, particularly in developing countries where rice is a staple food. Similarly, through precise edits, crops can be engineered to have a higher protein content or a better composition of fatty acids, directly enhancing the nutritional value of the global food supply.
In the context of climate change, the adaptability of crops to environmental stresses is paramount. CRISPR technology has been pivotal in developing crop varieties that can withstand extreme climatic conditions such as prolonged droughts, salinity, and fluctuating temperatures. For instance, crops are being engineered to enhance their water-use efficiency, allowing them to maintain productivity during periods of water scarcity. Additionally, efforts are underway using CRISPR to modify root architecture, enabling plants to access water and nutrients more effectively, which is crucial in less arable lands.
Another critical application of CRISPR in climate change adaptation involves the reduction of greenhouse gas emissions from agriculture. This can be achieved by developing crops that require less tillage, hence reducing carbon release from soils, or by engineering plants with increased photosynthetic efficiency, which translates into greater carbon capture and biomass production. Such innovations not only contribute to climate change mitigation but also ensure that crop productivity does not decline as a result of global warming.
A further application of CRISPR is the management of plant diseases and pests, which are expected to become more prevalent and unpredictable as the climate changes. CRISPR enables precise targeting and disabling of genes that make crops vulnerable to pathogens. This precision reduces the reliance on chemical pesticides, which are often harmful to the environment and human health. For instance, researchers have already made headway in enhancing the resistance of wheat to powdery mildew and of rice to bacterial blight, both of which are significant threats to food security.
Moreover, CRISPR technology is instrumental in the diversification of crops. Biodiversity in agriculture is a cornerstone of sustainability and resilience against pests and diseases. By leveraging CRISPR, breeders can develop new varieties more rapidly than ever before, ensuring that agriculture can keep pace with changing environmental conditions and consumer demands.
However, while CRISPR offers transformative potential, it also introduces challenges that must be navigated with caution. Ethical and safety concerns must be addressed, and thorough testing is required to ensure that CRISPR-modified crops do not have unintended effects on human health or the environment. Regulatory frameworks must evolve to provide clear guidance while encouraging innovation. There is a need for an inclusive dialogue that takes into account the views of all stakeholders, including scientists, policymakers, farmers, and the public.
As research progresses, upcoming breakthroughs may include the development of CRISPR-edited crops that can fix atmospheric nitrogen, reducing the need for synthetic fertilizers, and the design of perennial grain crops that can be harvested multiple times without replanting, reducing soil erosion and labor costs. The exploration of these and other innovations will depend on continuous advances in understanding plant biology, the evolution of CRISPR technology, and a commitment to addressing the ethical and practical considerations inherent in altering the genetic makeup of our food.
The ongoing evolution of CRISPR technology represents a critical component in the toolkit to achieve global food security. It offers an unprecedented opportunity to modify crops in ways that could not only increase agricultural productivity but also improve nutritional outcomes and adaptability to environmental changes. With careful stewardship, CRISPR has the potential to revolutionize agriculture, helping to ensure a stable and nutritious food supply for a rapidly growing global population amidst the uncertainties posed by climate change.
Future Developments and Innovations in Crop Engineering
The advent of CRISPR technology has introduced an exciting new chapter in crop engineering, fostering a wave of innovation that stands to dramatically reshape agricultural practices. As we look to the future, several promising developments loom on the horizon, each with the potential to further refine and expand the capabilities of this groundbreaking tool.
One of the most anticipated advancements in the field is the use of gene drives based on CRISPR technology. Gene drives are genetic systems that increase the chance of a particular trait being passed on to the next generation, well above the standard 50% probability. This method could revolutionize pest management in agriculture. For instance, gene drives could be employed to spread traits in pest populations that reduce their fertility or viability, thus controlling pest numbers in an eco-friendly and sustainable manner. This would reduce the need for chemical pesticides, which are known to have detrimental environmental effects, including the pollution of ecosystems and the harming of non-target species such as beneficial insects and pollinators.
Another frontier is the development of multiplexed gene editing, which allows for the simultaneous modification of multiple genes. This innovation can prove particularly beneficial in complex traits like drought tolerance and nutrient uptake, which are governed by an array of genes. Through multiplexed CRISPR, it may be possible to fine-tune these traits more effectively, crafting plants that can thrive in various climatic challenges and soil conditions, thus reinforcing food security in regions that are hardest hit by climate change.
CRISPR also harbors potential in the enhancement of photosynthetic efficiency. The ability to tweak the photosynthetic machinery of crops could lead to significant increases in yield, as plants could convert light energy into biomass more effectively. This area of research is particularly promising for food crops such as rice and wheat, which constitute the bulk of calorie intake for millions of people around the globe. The success of such modifications would represent a monumental leap forward in meeting the food demands of a burgeoning world population.
Furthermore, the continued refinement of base editors and prime editors offers a future where precise single nucleotide changes can be made without introducing double-strand breaks in DNA, which are typical of standard CRISPR/Cas9 editing. These tools increase the precision and reduce the likelihood of unintended off-target effects, elevating the safety profile of CRISPR-modified crops. This precision is essential not only for public acceptance but also for the adherence to regulatory guidelines that are becoming increasingly stringent.
Research into the creation of de novo domestication of wild plant species using CRISPR is another intriguing area. By inducing traits typical of domesticated plants, such as reduced seed shattering and increased fruit size, new crop varieties can be developed from wild ancestors. This could significantly diversify the types of crops available and introduce hardier varieties into the agricultural system, which could be vital for adapting to diverse environments and providing nutritional variety.
Synthetic biology, allied with CRISPR, also proposes revolutionary approaches, such as the development of synthetic chloroplasts that could be engineered to perform specialized biochemical reactions. For example, these could produce compounds that would make the plants more pest-resistant or even self-fertilizing plants that fix atmospheric nitrogen, slashing the reliance on synthetic fertilizers and lessening the environmental impact of agriculture.
A particularly exciting direction is the possibility of CRISPR technology enabling perennial grains. Perennials, unlike annuals, do not need to be planted each year, reducing soil disturbance, preserving topsoil, and maintaining carbon sequestered in the soil. This adaptation would have far-reaching implications for sustainable agricultural practices, particularly in terms of mitigating climate change and enhancing soil health.
The practical realization of these innovations depends on the continuous advancements in computational biology and genomics, as well. Algorithms that predict the outcomes of CRISPR edits with higher accuracy can streamline the development process. Meanwhile, advancements in genomic sequencing technologies can help identify the specific genetic variations responsible for desirable traits, providing a roadmap for targeted CRISPR interventions.
However, alongside these promising developments, a number of challenges persist that must be navigated to fully realize the potential of CRISPR in agriculture. The complexity of plant genomes and the polygenic nature of many important traits mean that simple single-gene edits may not always yield the desired results. The interaction of multiple genes and their expression in response to environmental stimuli can be highly complex, requiring sophisticated strategies for modification.
Scalability also presents a considerable hurdle. The transition from successfully edited crops in a laboratory setting to widespread cultivation in varied environmental conditions is non-trivial. It involves extensive field testing, regulatory approval, and eventually, acceptance by farmers and consumers. This process can be time-consuming and costly, impeding the rapid deployment of CRISPR-engineered crops.
Moreover, there is the challenge of ensuring equitable access to CRISPR technologies. The fruits of these scientific endeavors must benefit not just large, industrialized farming operations in developed countries but also smallholder farmers in the developing world, who are often the most vulnerable to the ravages of climate change and food insecurity. Addressing issues of intellectual property, licensing, and technology transfer will be key in democratizing the benefits of CRISPR-engineered crops.
Continued research is required not only to advance the science behind CRISPR and its applications but also to address these broader socio-economic and regulatory issues. As the scientific community moves forward, a multifaceted approach that combines innovation with sensitivity to public concerns, ethical considerations, and environmental stewardship will be essential to harnessing the transformative potential of CRISPR technology in agriculture.
Addressing Challenges and Limitations
As CRISPR technology continues to make waves in the agricultural sector, it is important to consider not just the potential benefits but also the challenges and limitations that come with its application. In the quest to make crops more resistant to diseases and environmental stresses, it’s crucial to understand the hurdles that must be overcome to ensure this technology can be fully utilized and beneficial for farmers worldwide.
Technological Limitations
While CRISPR has been hailed as a precise and versatile tool for gene editing, it is not without its technical constraints. One of the main challenges is the specificity of CRISPR-Cas systems. Off-target effects, where the Cas enzyme cuts DNA sequences that are similar, but not identical, to the target sequence, can lead to unintended genetic changes. These off-target mutations can potentially disrupt the function of essential genes, or cause undesired alterations that might affect the crop’s growth, yield, or nutritional quality. This is a particularly pressing issue in complex plant genomes where repetitive DNA sequences are more common.
Another limitation is the delivery method of CRISPR components into plant cells. Unlike animal cells, plant cells have rigid cell walls that make it harder for foreign genetic material to penetrate. Currently, methods such as agrobacterium-mediated transformation and biolistics (gene gun) are used, but they have their own drawbacks. For example, agrobacterium-mediated transformation is limited to certain plant species and can result in random integration of DNA, while biolistics can cause damage to plant tissues and also lead to random DNA insertion.
Unintended Consequences
The introduction of genetic changes, even when targeted, can result in unforeseen consequences due to the complexity of plant genetic networks. Altering a single gene might not only impact the trait it is directly associated with but can also affect other traits through genetic pathways and interactions that are not yet fully understood. Furthermore, gene editing can affect epigenetic regulation—changes that influence gene activity without altering the DNA sequence. This could lead to unexpected plant behaviors or characteristics that might not be evident until several generations later.
Scalability Challenges
Translating the success of CRISPR-based modifications from controlled laboratory conditions to the field is another significant challenge. The scalability of CRISPR technology faces several barriers, starting from the development of edited crops that must be rigorously tested in diverse environmental conditions to ensure their stability and safety. This process is time-consuming and expensive, often spanning several years. Scaling up also requires substantial investments in infrastructure and training for in-field testing and crop breeding.
Furthermore, because crop production involves complex interactions with the environment, successful lab experiments might not always yield the same results when scaled to actual agricultural settings. Factors like soil quality, climate variations, and ecological interactions with pests and other plants all play a role in the performance of CRISPR-edited crops outside of the lab.
Accessibility Issues
The uneven distribution of CRISPR technology’s benefits poses another challenge. Currently, the technology is more accessible to large-scale farming operations and developed countries that have the resources for high-tech agriculture. Smallholder farmers in developing regions, who could greatly benefit from disease-resistant or stress-tolerant crops, often lack access to such technologies due to high costs, intellectual property rights, and lack of infrastructure. Addressing this disparity is crucial for harnessing the full potential of CRISPR in global food security.
Intellectual Property and Regulation
Intellectual property rights surrounding CRISPR technology can also impede its widespread application in agriculture. Patents and licensing agreements may restrict the availability of the technology, making it difficult for researchers and breeders, especially in the public sector and low-income countries, to develop and deploy CRISPR-modified crops.
In addition, regulatory uncertainty can be a major roadblock. Different countries have varying regulations regarding genetically edited organisms, and the status of CRISPR-modified crops often falls into a grey area. In some regions, they may be regulated similarly to genetically modified organisms (GMOs), which are subject to stringent safety assessments and labeling requirements, while in others, they are treated differently due to the absence of foreign DNA in the final product. This inconsistency can create barriers to international trade and cooperation, as well as slow down the adoption and innovation of CRISPR technologies.
Addressing the Hurdles
To tackle these challenges, a multi-pronged approach is necessary. Researchers are continually working to improve the specificity and efficiency of CRISPR systems. Novel variations of the Cas enzyme and other CRISPR-associated proteins are being discovered and engineered for greater precision and minimal off-target activity. Additionally, alternative delivery methods that can safely and effectively transport the CRISPR components into plant cells are under development, such as nanoparticle-mediated delivery.
Risk assessment frameworks are also being refined to evaluate the potential impacts of CRISPR-edited crops comprehensively. These assessments go beyond simple genetic analysis to include ecological and long-term studies that can identify any unanticipated effects of gene editing. Moreover, efforts are being made to democratize access to CRISPR technology through open-source platforms, capacity building, and equitable licensing arrangements.
The challenges that CRISPR technology faces in transforming agricultural practices are significant, yet they are being met with dedicated research and innovative solutions. As the technology progresses, continued dialogue among scientists, regulators, farmers, and consumers will be essential to address the ethical, social, and environmental implications of gene-edited crops. Such collaboration will help in crafting policies that encourage responsible innovation while ensuring safety, transparency, and fairness in the global agricultural landscape.
With these combined efforts, the path forward for CRISPR technology in agriculture holds the promise of a future where crops are not only more resilient to disease and stress but are also accessible and beneficial for all members of the global community.
Conclusion: Integrating CRISPR into Sustainable Agriculture
Recognizing the transformative potential of CRISPR technology in agriculture invites both optimism and caution. The deployment of this revolutionary gene-editing tool in crop engineering heralds a new era of precision agriculture where scientists can fine-tune genetic attributes to bolster food production while simultaneously conserving natural resources. CRISPR’s ability to enhance crop resistance to diseases and environmental stresses stands as a testament to the power of human ingenuity in harnessing molecular biology for societal benefit. Yet, alongside these strides, we must navigate the nuanced landscape of ethical considerations, regulatory practices, and socio-economic implications that accompany the adoption of such technologies.
The ethical dimensions of CRISPR technology, particularly in the context of agriculture, delve into questions of biodiversity conservation, potential genetic contamination of wild plant relatives, and the long-term ecological impacts of altered crop varieties. While the technology provides a mechanism to rapidly develop crops that can withstand various challenges, ethical stewardship requires that these advancements do not compromise the ecological balances that sustain food webs and agricultural systems. The biosafety assessments and bioethical discourse must keep pace with technological advancements to address concerns surrounding gene drives and other gene-editing applications that could lead to irreversible changes.
As with any groundbreaking technology, the governance of CRISPR applications in agriculture is pivotal to its responsible development and deployment. The regulatory frameworks currently in place are a patchwork of national and international guidelines that reflect a diversity of viewpoints on genetically edited organisms. These regulations must be agile enough to adapt to the rapidly evolving landscape of CRISPR technology while remaining stringent enough to safeguard public health and environmental integrity. It’s a delicate balance between fostering innovation and enforcing oversight, one that requires the input of scientists, policymakers, and stakeholders from across the agricultural spectrum.
The dialogue around CRISPR must also contend with public perception. Transparent communication regarding the capabilities, risks, and benefits of CRISPR-edited crops is necessary to build public trust and informed consent. This is especially critical when addressing the concerns of consumers who are wary of genetically altered foods. Educating the public on how CRISPR differs from traditional genetically modified organisms (GMOs), and how it may be used to advance organic and sustainable farming practices, can bridge the informational divide and dispel misconceptions.
Continued innovation in CRISPR technology promises to unlock further agricultural possibilities. Advances such as base editing and prime editing expand the toolkit available to scientists, providing even more precision and versatility. These innovations could enable the targeted activation or repression of genes, allowing for more nuanced control over plant traits. The ongoing quest for more effective delivery systems for gene-editing components into plant cells also highlights the innovative spirit driving the field forward.
Collaboration will be central to the success of CRISPR technology in agriculture. The pooling of intellectual and material resources among academic institutions, industry, and international organizations is crucial for accelerating the pace of discovery and application. Partnerships that extend across borders can facilitate the sharing of CRISPR-edited crops that are tailored to local conditions, particularly benefiting regions that are most vulnerable to climate change and food insecurity.
While the evolution of CRISPR technology continues at a breakneck pace, so too must our collective efforts to ensure equitable access. Addressing the disparities in technology distribution is fundamental for realizing CRISPR’s full potential in agricultural transformation. Equity in access entails not only the dissemination of CRISPR tools and techniques but also the empowerment of smallholder farmers through training and capacity building. By cultivating local knowledge and expertise, CRISPR technology can be integrated into diverse agricultural practices, fostering resilience and sustainability at the grassroots level.
The journey of integrating CRISPR into sustainable agriculture is an ongoing one, where the interplay between discovery and deliberation shapes the trajectory of food systems. As we forge ahead, the commitment to rigorous scientific research, inclusive policy-making, and ethical foresight will be the cornerstones of progress. It is through the responsible application of CRISPR technology that agriculture can reconcile the demands of a growing global population with the imperative of ecological stewardship, setting the stage for a future where innovation nurtures both the land and those who depend on it.